MEETING # 1621
4:00 P.M.
October 7, 1999
Bridges
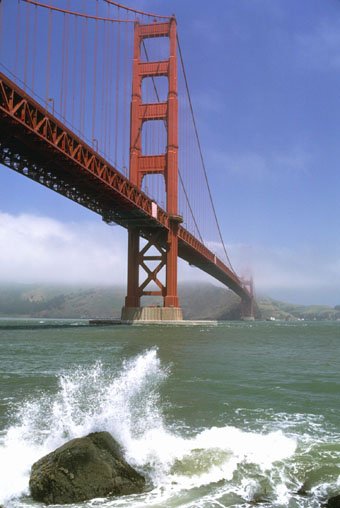
by John Templeton
Assembly Room, A. K. Smiley Public Library
SUMMARY
From ancient times humans, with ever increasing ingenuity, have found ways to cross waterways and chasms. From fallen logs, to carefully placed stepping stones across a stream, to stone and iron-truss structures, to the engineering marvels of todays suspension bridges there is almost no obstacle that mans ingenuity cannot overcome when commerce dictates a crossing.
Background of the Author
John A. Templeton was raised in Mt. Lebanon, a suburb of Pittsburgh, Pennsylvania. He received his B.S. from Worcester Polytechnic Institute in 1945 and his M.S. from the University of Maryland in 1951, both in Mechanical Engineering.
He served in the U.S.Navy in 1944-45 and was then employed by the Naval Ordnance Laboratory in Washington D.C. and White Oak, Maryland engaged in the design and development of bomb and rocket fuzes.
In 1955, as an Operations Analyst on the staff of Gen. Curtis LeMay in Omaha, Nebraska, he developed techniques for delivering thermonuclear weapons from Strategic Air Command B-36 and B-47 bombers.
After several years with the Magnavox and Avco companies, the family moved to California, first to Palos Verdes Estates and then in 1962 to Redlands. John was employed by TRW for some thirty years engaged in the systems engineering and technical direction of the Minuteman and MX ICBM programs.
He and Margie have enjoyed almost fifty five years together. Their four children reside in Wellesley, Massachusetts, Corona Del Mar, Costa Mesa, and Redlands, California. Their nine grandchildren span almost a generation-from the oldest at 31 to the nine year old youngest.
Contents
Prehistoric Construction
Structural Principles
The Suspension Principle
Stone Arch Construction
Iron Girder Construction
John Roebling and the Brooklyn Bridge
The Golden Gate Bridge
Galloping Gertie
The Future
Prehistoric Construction
The use of bridges is as old as mankind. Over millennia the simple hanging rope of prehistoric times developed into elaborate suspended structures of bamboo and iron; crude stepping stones were superseded by mighty pontoon bridges of cabled-together ships, and simple wooden spans were overshadowed by the mighty stone arches of Rome.
Many of our earliest hunter-gatherer ancestors must have had to range far and wide in their quests for food , fuel or shelter; and inevitably they must have encountered natural obstacles such as streams, rivers and chasms. To cross from one side to the other, for whatever purpose, casual use of what nature and chance threw their way had to come before any deliberate emplacement.
Where running water eats away soft strata or shale from beneath more durable layers of rock, natural stone arches can be formed, and perhaps bridges such as these were used by some of our forebears. Some of them were, and indeed are, enormousfor example, American Indian folklore tells of the mighty Tomanowos ("bridge built by the gods"), which once spanned the Columbia River between Washington and Oregon. It fell centuries ago, but the rubble is still said to be visible on the river bed. Today, the longest natural rock spans still surviving are the Landscape Arch and the Rainbow Bridge in Utah, both spanning over 203 feet.
Infinitely more often, though, it must have been dead tree trunks or branches, conveniently toppled across gaps, which formed the first bridges for the feet of our remote ancestors, ages before their descendants contrived to put in place what chance and nature had not provided. And how often did primitive man grasp a hanging jungle vine and swing out over a narrow rushing stream, unaware that millennia later the same fundamental interaction of materials and forces would be used to hang immense steel ribbons across incomparably vaster watercourses?
With the development of stone tools, crude at first but increasingly efficient as time passed, it was perhaps not too much of a collective mental leap for a particularly perceptive tribe to grasp the idea of hewing down a tree-trunk with flint hand-axes and dragging it across the narrowest width of an inconvenient stream, rather than search up and down the banks for shallows or wait for a log to fall into place of its own accord. It was then only a short step to felling several trees and laying their trunks side by side to make a broader surface, and then to lashing them to one another, so that the bridge held together. Then, rather than use whole trunks, why not split the timbers to form rough flat planks, both to make the bridge lighter and the crossing smoother? Downstream, perhaps, skills were progressing as far as laying smaller branches of a similar length transversely across the space between two long separate edge trunks, to form a wider and flatter bridge floor; while up in the hills, where, perhaps, faster-flowing waters were forced between narrow-sided gullies under overhanging rock ledges, others may have been bridging the gap that remained with a couple of slabs of rock propped against each other in an inverted "V", or , if it were long enough, with a single slab.
Sometimes the river would have been too wide to have been spanned by single beams, whether they were the timbers plentifully available in temperate parts of the world or the stone slabs of the harsher regions. In either case, however, if some conveniently flat rocks were at hand, and if the water was shallow enough, the rocks could be dragged in to form mid-stream stepping stones; and if the water was too deep for this, they could be piled up to make primitive piers, on which the ends of the beams could be balanced from the shore. Again if the river-bed was suitably soft, it might have been more practicable to make the piers out of timbers or long stone slabs rammed in end-wise and buttressed at the sides, rather than crudely stacked.
Any such sequence of events must be speculative: these advances undoubtedly happened, but piecemeal. One thing is certainthe development of bridge-building skills was already well under way before the earliest era of recorded history.
Of course, no original prehistoric bridges are known for certain to have survived, but in many parts of the world there are still examples of types so basic that for all intents and purposes they can be regarded as such. In England, the timber "clam" bridges of south-east Cornwall and the stone "clapper" bridges of Dartmoor would seem to be examples of prehistoric construction technologyand who can be absolutely certain that some of these ancient granite slabs are not the very same stones as were trod by our remote forebears?
Structural Principles
Every bridge ever built, from the first log across a stream to the latest gravity-defying marvel, has used the properties of its materials to withstand, overcome and exploit the forces to which it is subjected. These forces have three origins: the bridges own weight, the dead load; the traffic passing over it, the live load; and other external environmental loads, such as wind, earthquakes or water.
The combinations of different forces and the different strengths of materials produce varying effects in the basic types of bridge. On a simple beam, such as a log or a stone across a stream, the combination of its dead load and any live load results in bending; it experiences compression on its top edge and tension along the bottom. The tensile strength of a hardwood tree-trunk allows it to take a good deal of both forces before it breaks, but if a stone beam of similar proportions is subjected to the same loading, it will not bend to anything like the same degree before it cracks at its bottom edge.
In the second type of bridge, the arch, stresses are in compression, as dead and live loads combine to press the material together. The arch form is thus ideal for construction in stone, because the materials compressive strength is used, while its lack of tensile strength becomes irrelevant.
The third basic bridge type, the cantilever, is, in essence, a bracket secured at one end with the other end hanging free. The simplest form of cantilever bridge has a central beam supported between opposite cantilevers. So-called natural stone bridges are in fact closer to being cantilevered.
It is important to consider how the structures interact with the ground. In beams the only forces acting on the ground are vertically downwards. The same is true of centrally supported cantilevers, but arches tend to push outwards as well as downwards, and so need secure abutments to prevent them from sagging and eventually collapsing.
The Suspension Principle
The beam and the cantilever are not the only bridge types to have evolved long before the dawn of history: different climates, terrains and materials led to different methods of spanning space. A single rope or cable across a chasm is also effectively a bridge. In fact it is the most primitive form of another basic bridge type, the suspension bridge.
Primitive forms of the suspension bridge are still in use all over the world. In remote parts of India there are examples as long as 660 feet which consist of nothing more than a twisted bamboo rope. Travelers slide down it hanging from a bamboo loop, and when gravity gives out, they pull themselves along the remainder of the rope hand-over hand. In a version once found in China, travelers carried their own wooden saddle with a deep groove cut into the underside. On reaching a single strand bridge, they fitted the cable into the groove, mounted and let gravity do the rest.
In the 1930s an expedition to the then Belgian Congo filmed the construction of a bridge by a group of pygmies. First, a rope was suspended almost to the ground from the top of a tall tree overhanging the watercourse. Then a small boy clung to the hanging end and was swung further and further out until he was able to grasp a branch high up in a tree on the opposite bank. Once he had secured his rope and clambered back the first rope was used to carry over others. After only six days, an elastic air-borne half-tube, 165 feet long, had been woven. Inca constructions, some over 200 feet long, had several massive cables, bound together, supporting a flat assembly of planks.
Stone Arch Construction
Technology has always been the driving force of innovation in bridge building and design. The Romans made the first leap forward in building materials, changing from timbers to more durable stone. The best structural form that could support stone was the half-circle arch. The original innovators of this arch seem to have been the Etruscans who used wedgelike, tapered stone blocks, called voussoirs, to construct their arches. Afterwards, the half-circle arch bridge was used effectively by the Romans for the next five centuries. Many of these bridges are still standing.
The greatest construction problem facing the Romans was building a suitable foundation to support the weight of the stone bridge and the traffic going across. Earlier civilizations built bridges by temporarily diverting the river and working directly on the dry river bed, or by dropping stones into the water until they massed on the bottom of the river bed, or by utilizing available rock outcroppings to construct bridge piers.
The Romans, however developed cofferdams: timber piles were driven into the river bed to form a box where a bridge pier was to be located; the water was then drained out to create a dry enclosure suitable for building purposes This method resulted in bridges that have stood for centuries and enabled the Romans to construct foundations in the middle of practically any waterway.
The most important Roman bridges were built of stone, with stone piers, stone roadways, or both. One famous example, constructed of stone piers with a timber span, is Trajans Bridge, which was built over the Danube in A.D. 106. Engraved on a surviving stone pier is the following inscription: "The Roman Augustus, truly a great bridge builder, constructed this bridge that even the grand rapids of the mighty Danube cannot overcome." Unfortunately this inscription did not save Trajans Bridge from the hands of the Romans themselves. I order to prevent invaders from using the bridge, the Romans destroyed the timber spans less than thirty years after their construction.
The strength of Roman piers was the result of a closely held technological secret: a natural cement named pozzolana. When mixed with lime, this volcanic sand creates a hydraulic cement comparable to modern-day concrete. It was used in foundations and piers submerged under water. The Romans were also fine brick makers, and they manufactured large, flat, clay, earth, and sand tiles. These bricks were dried for long periods to create a material that was both durable and structurally sound.
In building their half-circle arches, the Romans built on a grand scale, with spans extending more than 130 feet. The half-circle shape meant that each voussoir was exactly the same shape. These arch stones, which required no mortar between them, sometimes weighed more than seven tons and often needed to be raised more than 100 feet into the air, then supported and secured with the next stonea daunting task.
The emperor Hadrian, an amateur architect and bridge builder, left an enviable legacy. To control trade and enforce their rule in the regions they had conquered across Europe, the Romans built walls in Great Britain, Germany, and Rumania. Around A.D.120 they constructed a stone wall that measured ten feet wide and twenty feet high and extended 73 miles from the Tyne River to the Solway Firth in Great Britain. There is evidence that this wall, called Hadrians Wall, actually crossed over the Tyne on a Roman bridge made of timber.
Spain is also the site of many impressive bridges built by the Romans. The Alcantara Bridge over the Tagus River was built during the reign of Emperor Trajan. The structure is still standing after nineteen centuries and is prominently featured in Spanish travel brochures. When the French Grande Armée retreated across the bridge in 1812, they destroyed one of the larger spans in an effort to halt the advance of the Duke of Wellingtons army. The strategy did not succeed , however, and the pursuing army was able to cross over on a hastily constructed suspension bridge made of rope. In rebuilding the war-ravaged bridge, the Spanish had to resort to the use of mortar, a practice the Roman engineers had never had to follow.
Iron Girder Construction
The second great leap forward in bridge building would not occur until the Industrial Revolution of the 18th century. A major impetus was the newly invented railroad, which created the need for bridges that could span rivers and carry the heavy loads of steam engines and their freight. The first bridge made of cast iron was a semi-circular arch supporting a roadway constructed over the Severn River in Coalbrookdale, England, in 1779.
Not long after iron began to be used, its strength and value became clear to British engineer Thomas Telford. His structures spanned rivers without the need for intermediary piers in midstream. This wide-span construction prevented damage brought on by floods and ice and offered boats clear sailing under the spans.
In 1800 Telford submitted a bridge design to replace the crumbling and ancient London Bridge. Had it been built, Telfords 600 foot cast-iron span would have captured the worlds attention eighty years ahead of Eiffels tower or Roeblings Brooklyn Bridge. Instead Parliament approved a stone masonry bridge design. Iron bridges were relegated to rural locations where stronger structures and longer spans were required for the burgeoning railroads.
In 1826 Telford completed one of his masterpieces, the bridge over the Menai Straits in Wales. The span of 580 feet was the worlds longest suspension of its day, and the forerunner of modern bridge design. Thomas Telfords innovative bridge concepts established him as the father of the iron bridge. His concern for aesthetics, his use of new materials, and his structural daring set him apart from other structural designers of his day.
The age of the iron bridge culminated in the latter part of the 1860s with the creative geniuses of Gustave Eiffel and John A. Roebling. Eiffels fame has endured and grown with the stature and elegance of the Parisian tower named for him. Its construction is the same structural design he had developed for his bridges and viaducts.
John Roebling and the Brooklyn Bridge
The most prominent nineteenth-century American structural engineer was John Augustus Roebling, the father of the modern-day cable suspension bridge. Roebling was a man of vision who established a wire rope manufacturing plant to fabricate the wire cable he would utilize for his suspension bridge designs.
Roeblings first suspension bridge was built over the Allegheny River in 1844, followed by a roadway span in Pittsburgh, PA over the Monongahela River. Roeblings first widely acclaimed structure was his combination road-and-rail suspension bridge over Niagara Falls.
Initially, in the late 1850s, the citizenry of New York City had rejected Roeblings urging to span the East River with a suspension bridge. However, the bitterly cold winter of 1866 changed the minds of the residents of both cities bordering the East River. The ice-choked river prevented ferry commuters from getting to work, and the campaign to build a bridge across the river finally gained civic support. By the time the Brooklyn Bridge was opened to the public on May 24, 1883, the bridge had gained a symbolic national and international importance, and had single-handedly ushered a new technological era.
Roeblings dream was to build the longest suspension bridge in the world. He wanted to build a "Great Bridge" with a clear span across the East River without any intermediary piers in midstream. This would require a span of close to 1600 feet, a distance never before attempted. Roebling envisioned two bridge trains on a continuous cable carrying passengers over the bridge in less than five minutes. On the same deck, on either side of the trains, two vehicular lanes would permit wagons and carriages to travel in each direction. Above the trains an "elevated promenade" would provide a way "for people to stroll across the bridge on fine days in order to enjoy the beautiful views and the pure air."
Roeblings vision included twin towers, each with a pair of Gothic arches one hundred feet high. His proudest achievement would be to suspend this magnificent and daring structure with steel wire cables and to tie everything together with steel inclined stays and steel vertical suspenders.
The two massive stone towers, each weighing over sixty-seven thousand tons, had to have firm and solid foundations. These colossal towers, each rising to a height of 276 feet would be taller than any existing buildings in the area and would thus dominate the skylines of both New York and Brooklyn.
Before this visionary engineer had the opportunity to see his towers rise, he died as the result of a freak accident while surveying for the towers. Trying to avoid the impact of an oncoming Fulton Street Ferry on the East River pier on which he was standing, Roebling caught his foot between a rope and the timbers of the pier, resulting in the amputation of several toes. While the amputation didnt kill him, his attempt at home remedies did. Thinking he could cure himself, he continually ran cold water over his wound, despite the objections of the many doctors whom he continued to dismiss and replace. His wound festered and tetanus set in, resulting in lockjaw. Roebling suffered immeasurably over an extended period of time, experiencing terrible pain. He died before the completion of his masterpiece.
Roeblings thirty-two-year-old son, Colonel Washington A. Roebling, who had been assisting his father with the project was promoted to chief engineer and undertook completion of the work. He devised a pressurized timber-, tin-, and ironclad caisson that would support the twin towers. As the excavations continued, the pneumatic caissons, which were the largest in the world, were slowly sunk into the soft riverbed. Washington Roebling described the caissons as "diving bells with open bottoms that formed an airtight pressurized work chamber. Workers descended into the caissons through airlocks in order to dig out the soft riverbed, thus enabling the continued lowering of the caissons by the massive stone weight being placed upon them. In this way, the caissons eventually reached a solid rock foundation where they could be anchored and filled with concrete.
One obstacle was the debilitating condition then called "caisson disease" (now known as the bends), an affliction unknown at that time, as no other construction project had required divers to go so deep in a riverbed. This condition, it was later learned, resulted from too-rapid decompression experienced after exiting from the high pressure of the caissons.
Eventually, Washington Roebling was himself to succumb to a crippling and disabling paralysis as a direct result of caisson disease. He was forced to watch the ongoing construction through field glasses from his Brooklyn Heights window. Under his direction, his wife, Emily Warren Roebling and his assistants completed the bridge in fourteen years. The Brooklyn Bridge was formally dedicated and opened by President Chester A. Arthur on May 24, 1883. It was one of the greatest engineering feats of the nineteenth century and one of the most inspiring.
John Roebling had predicted that the clear span of suspension bridges could be more than twice that of the Brooklyn Bridge. His prophecy became a reality in 1931, when the George Washington Bridge spanned the Hudson River with a clear span of thirty-five hundred feet. At night, the reflection of the cable lights dancing on the Hudson River, shimmering like a string of pearls gently descending from the tips of the towers, is breathtaking. To anyone sailing or driving up the Hudson River at dusk, the magnificent panorama of the stately George Washington Bridge presents itself between the high bluffs of the Palisades on the New Jersey side and Washington Heights on the New York shore.
The Golden Gate Bridge
There was no better opportunity for surpassing the George Washington Bridge than on the west Coast, where gale-whipped ocean currents surged through the often fog-blanketed mile of the Golden Gate between San Francisco and the Marin Peninsula. Long discussed, the idea was not taken seriously until 1917, when Joseph Strauss proposed a design for a vast and rigid cantilever/suspension hybrid. But during the ensuing decade of administrative wrangling, the George Washington demonstrated the pre-eminence of suspension structures for the longest spans.
Although Strauss remained in charge, a new design was drawn up, principally by his chief assistant, Charles Ellis. The weather and risk of earthquake necessitated rigorous structural safety; the heavy shipping traffic demanded a deck of unprecedented height for clearance; and the glorious site invited a design of corresponding beauty. The towers were elegant pairs of vertical shafts, stepped back three times and linked at these points as well as at the top by deep horizontal beams. Both step-height and beam-depth diminish as the towers rise, enhancing the bridges grace. They sail more than 700 feet into the air. The suspended deck, 700 feet longer but much narrower than the George Washingtons needed a stiffening truss, but it was shallower in relation to the great span than any yet achieved.
Work began in January 1933 on the shore anchorages. The north pier was built relatively easily on a bedrock ledge only 20 feet below water, in a coffer dam against the Marin cliff. But on the San Francisco side, the best option was 1100 feet from shore, in water 100 feet deepvirtually the open ocean, amid frequent shipping. Engineers finally decided on a cofferdam designed foundation and poured sixty-five feet of concrete into it to complete the foundation.
By August 1935 the towers had been erected and the cable spinning operation began. The following fall saw the deck sections built out from both sides of each tower simultaneously, in order to balance the weight on the cables.In an extremely heavy gale force wind of 120 miles per hour the bridge was calculated to sway more than twenty feet (deemed acceptable by the engineers in charge). On 27 May, 1937 pedestrians200,000 of themwere allowed on the completed bridge, and on the morning of the following day it was opened to traffic.
Galloping Gertie
It was Leon Moisseiff who designed the ultimate in slender suspension bridgesand one which within four months of its opening in July, 1940, became the most notorious. The Tacoma Narrows Bridge over Puget Sound had a suspended span of 2800 feet, was only 39 feet wide, and had a structural weight only a small fraction of the George Washington Bridge. It was promptly nicknamed "Galloping Gertie" because of its motion under quite light winds, which not only caused it to sway from side to side but also sent rippling waves along the deck.
On 7 November, 1940, a moderate wind of around 42 mph set up a severe lateral twisting of the deck as well as longitudinal rippling. There was a cameraman present to film Galloping Gerties death-dancehundreds of tons of writhing steel tearing themselves loose from their anchorages and plunging to destruction.
A reporter from the local newspaper had driven to the center of the span that morning to get a first-hand description. His daughters small dog was along. The wild whipping of the roadway became so violent that he was barely able to return to safety by crawling on hands and knees while his car slid from side to side. The dog was the only casualty; the reporter wondered how he would explain its death to his daughter.
The Future
There have been remarkable advances in suspension spans in the last half of the twentieth century. In France, the Pont de Normandie across the mouth of the River Seine is a cable-stayed structure completed in 1994. In England, the bridge across the Humber Estuary set a new world record for length in 1981¾ 4,624 ft.
Intercontinental spans? The Bosporus between Asia and Europe has been bridged several times, but other possible locations for intercontinental bridges are vastly more challenging and forbidding. Europe to Africa between Spain and Morocco has been seriously studied as has an Inter-Continental Peace Bridge linking Siberia and Alaska.
In the final analysis bridges are built from need. They are far more than material connections between two points of land. They also serve as links between societies, cultures and political ideologies.
Bibliography
Landmark American Bridges by Eric DeLony
Library of Congress Catalog Card No: 92-24530
Bridges by David J. Brown
Library of Congress Cataloging-in-Publication Data
Bridges by Steven A. Ostrow
Library of Congress Cataloging-in-Publication Data
Bridges by Judith Dupre
Library of Congress Cataloging-in-Publication Data
|